11-minute read
Quick summary: Advancements in green hydrogen energy production and infrastructure are making it a promising clean-energy solution for hard-to-electrify sectors, transportation, and utilities, paving the way for a sustainable future.
Hydrogen energy has earned its share of attention over the last few years and decades, with opinions varying widely from “Hydrogen is a small niche player in the energy market” all the way to “Hydrogen is the world’s first choice for everything energy.” As in most cases, the truth likely lies somewhere in between these two extremes, and over the next few years, the scope of that truth will become increasingly clear.
What we can say for certain is that the momentum for hydrogen is at an inflection point. Countries around the world are crafting ambitious hydrogen strategies, investing in infrastructure, and fostering international collaborations to create a robust hydrogen economy. Investments are streaming into innovation in the three “pillars” of the hydrogen value chain: production, transport, and utilization. Competition for the diverse solutions to the clean energy revolution is constantly heating up, and hydrogen has a role to play. But what is that role, and how influential will it be?
In this article, we’ll explore where hydrogen energy came from, where it stands today in terms of production, transportation/storage, and utilization, and the compelling use cases that point to a bright future for this powerful energy source.
A brief history of hydrogen energy
The most abundant element in the universe has long captivated scientists and innovators with its potential as an energy carrier. The mid-20th century saw hydrogen’s potential as an energy carrier begin to take shape when, during the space race, NASA adopted liquid hydrogen as a rocket fuel, demonstrating its high energy content and clean-burning properties.
This period also marked the development of fuel cell technology, which converts hydrogen into electricity with water as the only byproduct, rendering techniques to use hydrogen proven and laying the groundwork for it to become a player in the energy space. However, widespread adoption remained limited due to technological and economic barriers, as the cost of hydrogen due to production efficiency challenges and transport infrastructure was extremely high.
The hydrogen color spectrum
Hydrogen is a unique form of energy because it is rarely produced naturally and instead needs to be manufactured, resulting in low round-trip efficiency for power use. Modern Lithium-ion batteries have roundtrip efficiencies close to 80 percent, meaning that 80 percent of the energy that was input into the battery remains after charging and discharging losses. Hydrogen, on the other hand, has almost half that round-trip efficiency due to the need for electrolysis, transport, and finally energy creation in a fuel cell. However, there are multiple use cases where hydrogen becomes much more attractive, including long duration energy storage (LDES) and hard-to-electrify manufacturing processes (see “Top use cases for hydrogen energy” below).
Hydrogen is almost always referred to by its “color,” a way to describe how it was produced and the emissions embedded alongside that production. The three most common colors are grey, green, and blue:
- Grey hydrogen: Produced from natural gas through a process called steam methane reforming (SMR). This method emits significant CO2, contributing to greenhouse gas emissions.
- Blue hydrogen: Also produced from natural gas, but with carbon capture and storage (CCS) technology to capture and store the CO2 emissions. This results in a reduced environmental impact compared to grey hydrogen.
- Green hydrogen: Generated through electrolysis of water using renewable energy sources like wind, solar, or hydroelectric power. This method produces hydrogen without any CO2 emissions, making it the most environmentally friendly option.
A decades-long push-and-pull with hydrogen has made it constantly both on the cusp of taking over energy and also being mostly silent as wind, solar, and batteries have taken precedence. As hydrogen has stubbornly held on to its expensive price tag from decades before, other players in the clean energy landscape have dramatically declined in cost. Nonetheless, hydrogen still has a role to play in the energy transition. Those same cost reductions in wind and solar have meant lower green hydrogen prices, and there are multiple use cases where hydrogen is either the only solution available or a very competitive one. Diversification of energy solutions is the most robust and reliable way to design a carbon-free energy future, and hydrogen certainly has a powerful role to play in the energy transition.
Hydrogen production: The future is green
The current state of hydrogen production is marked by significant advancements and a growing emphasis on sustainability, yet numerous challenges must be addressed if it is to fully realize its potential as a clean energy source. As over 90 percent of the current hydrogen energy is produced by polluting methods, there is considerable interest in moving towards green hydrogen to help countries realize their net-zero goals.
A green hydrogen production plant consists of a row of electrolyzers that produce the hydrogen and a mixture of renewable energy generation sources and energy storage. The plant produces clean power, which is used by the electrolyzers to create hydrogen with no associated emissions, with the storage installation used to smooth power output from the wind and solar or to power the electrolyzers during times of little or no renewable power.
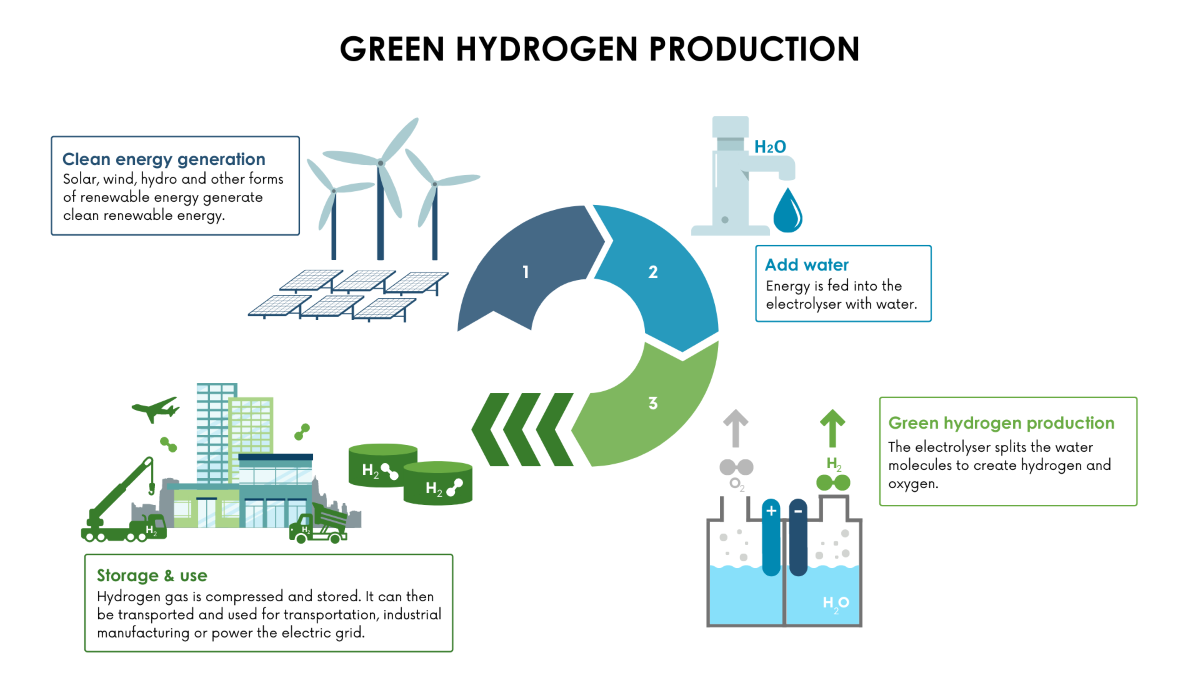
(Image source: New Jersey Department of Environmental Protection)
The challenge of staying in the “green” zone
There is a looming cloud over green hydrogen production, however, and that is its ability to truly stay “green.” Two main types of hydrogen production plant design exist. The first is fully disconnected from any nearby grid and uses its own clean energy, in essence becoming its own micro-grid. The second is a facility that is connected to a larger grid and can import and/or export power to and from this larger market.
The fully disconnected plant has no problems with proclaiming itself green, but when plants rely on a nearby larger grid to provide stability and supplemental power support, it’s difficult if not impossible to ascertain exactly where that energy was produced. For green hydrogen plants to be transparent with their emissions and prove the hydrogen is not simply being supplemented by natural gas power from the larger grid, there needs to be an accountability and paper-trail process that is currently non-existent.
To make this matter even more complex (and important), the recent Inflation Reduction Act established section 45V to provide a production credit for clean hydrogen in order to offset high capital costs of these projects. Credits are tiered based on how much emissions were associated with the hydrogen production, with the credit for the cleanest hydrogen at $3/kg. As the cost of green hydrogen currently sits around $5/kg, this credit represents a significant cost reduction that can bring H2 within the realm of cheaper fuels and make it economical for larger plants and projects. The successful implementation of this credit could mean life or death for some green hydrogen projects, so it is paramount to establish robust and straightforward emissions reporting for the hydrogen production lifecycle.
Hydrogen storage and transport
Cost reductions are the only way hydrogen can become competitive with other energy sources, and the unique challenges of transporting and storing hydrogen make this one of the most promising areas where large price mitigations can occur. It’s difficult to hold hydrogen in a cheap and reliable way because it is highly flammable and easily leaks from tanks and pipes. According to Argonne National Laboratory, around 80 percent of the cost of hydrogen is contained in the transport and storage methods. With significant capital needed for safe transport, this is clearly a high-potential target area to reduce the cost of hydrogen.
A mixture of transport and storage methods are used today, each having its own reasons for being used in different locations or situations.
Transporting hydrogen in liquid form
One of the biggest problems with hydrogen transport is that as a gas H2 takes up lots of volume and returns relatively low energy potential. This means the more H2 you can pack into a space the better, and this is where keeping hydrogen in its liquid form becomes attractive. To do this, you need to cool H2 to below -240°C and keep it there, all the while maintaining a balance of pressure and temperature to reduce boil-off of your now-liquid H2. In a very energy-intensive process, the hydrogen needs efficient heat exchangers and well-insulated tanks to keep it cool as it’s being stored, resulting in high costs for the cooling infrastructure needed. This high cost is somewhat offset by the ability to transport large amounts of H2, which makes H2 transport advantageous for medium-distance transport and for applications requiring large amounts of the substance.
Transporting hydrogen in gaseous form
Transporting hydrogen in its gas form is enticing because you don’t need to expend excess energy cooling it into a liquid or combining it with another substance. However, the low volumetric energy density problem persists and requires hydrogen storage at high pressure to fit as much of it in a tank as possible. Specially designed high-pressure tanks are used to efficiently squeeze in as much H2 as possible and hold it safely. Depending on the pressure needs of the tank, various materials are used ranging from relatively cheap but heavy steel to the more expensive but lighter carbon fiber. A slight reduction in overall cost from the lack of thermal energy compared to liquid H2 makes gaseous hydrogen transport economical for short-distance transport.
Other hydrogen transport and storage methods
Two other promising storage techniques are using underground salt caverns and attaching hydrogen to another substance to in effect “carry” the hydrogen. Salt caverns reduce costs immensely due to the lack of need for complex storage tanks to pressurize or cool the hydrogen, but obviously are limited by natural variability. There are only a handful of these caverns that would be suitable in the United States, and therefore limit the total scope of this storage measure.
Liquid organic hydrogen carriers (LOHCs) are more promising. A strong potential candidate for this is ammonia (nitrogen bonded with three hydrogen atoms), where through hydrogenation with the air ammonia is created and can “store” the hydrogen within its own bonds. This allows transport companies to utilize existing ammonia infrastructure due to the less volatile and less challenging nature of ammonia. However, the common thread of efficiency losses is unfortunately still present here, as it takes energy to both add hydrogen to a carrier substance and subsequently “crack” it back into pure form again.
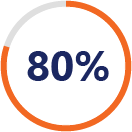
Top use cases for hydrogen energy
So why use hydrogen at all? There are a few specific use cases for hydrogen that make it an exciting innovation for the future of the energy landscape. If cheap green hydrogen and an increase in infrastructure can become mainstream, fuel costs will become lower than today’s popular choices of gasoline and diesel. The big question is if this can happen and why countries should opt for spending billions on developing new hydrogen infrastructure instead of other options.
If businesses develop and mature some of the use cases outlined below, hydrogen infrastructure can be built to satisfy necessary applications now and lay the path for cheaper costs down the line. There are three broad areas where hydrogen can make the most inroads versus other competitors, namely hard-to-electrify sectors, transportation, and utilities.
Hard-to-electrify sectors
The most crucial utilization of hydrogen to reduce carbon emissions will be in the manufacturing sector. These are current use cases for hydrogen where it can’t be substituted for anything else and the process can’t be electrified to tap into the increasingly green grid. Agricultural fertilizers, steel refining, and petroleum refining all need large amounts of pure hydrogen that right now is produced cheaply with steam methane reforming as grey hydrogen. This represents a huge potential for emissions reduction simply by replacing grey hydrogen with green hydrogen, in an industry that is already set up to deal with the challenges of moving and storing hydrogen.
Transportation
Using fuel cells to power cars and trucks offers many advantages over gasoline, most of which are shared with battery electric vehicles (BEVs), making them the biggest competitor in this space. Over the last 15 years, large investments in BEVs have made them far more popular than the few hydrogen cars on the market, and in small/commuter applications, hydrogen cars lag in the infrastructure battle with BEVs and continue to fall behind in sales and manufacturing scale year over year.
As vehicles increase in size, providing sufficient power requires larger and larger batteries that are also extremely heavy. This tips the scales back in favor of hydrogen, where quick fueling times and lighter power train needs mean that long distance cargo transport by hydrogen trucks is still an intriguing solution.
Shipping has a similar story; the larger the ship, the more cost effective a hydrogen solution becomes. Between using hydrogen fuel cells and burning hydrogen in combustion engines as a clean fuel, the shipping industry is facing large calls for decarbonization, of which hydrogen can become a key solution.
The key limiter to using hydrogen in widespread transportation applications is cost (sound familiar by now?). If hydrogen can’t beat out the cost of diesel for heavy transport, it simply cannot compete with other decarbonization options like batteries. However, hydrogen use cases in manufacturing as shown above can provide a roadmap for infrastructure development that may offset some of the high cost of development.
Utilities
There are many different energy storage technologies that are trying to fill the gap left by intermittent generating sources like wind and solar. While falling costs of batteries have put them in position to tackle short-term storage needs (under 12 hours), lithium-ion becomes much more expensive in cost per kilowatt-hour storage capacity when storage is required for days or weeks.
This is where hydrogen can again enter the ring, and since hydrogen can be stored indefinitely until use, it can be used for long-duration energy storage. For grid-level storage needs, an in-depth analysis is needed to evaluate which technology can improve reliability and bring down costs of renewable generation plants, and having hydrogen as a viable alternative to batteries will be hugely beneficial to project developers.
A promising piece of the clean energy puzzle
The story of hydrogen has many familiar themes: lots of potential and excitement for a world-changing substance and key limitations that have dampened those expectations for years. As the clean energy ecosystem continues to take shape, it’s clear that hydrogen will play a key role, particularly in utilities, transportation, and hard-to-electrify industries. Fueled by falling renewable energy costs and government programs, green hydrogen production is slowly gaining steam. As collaboration across industries unlocks hydrogen’s potential, a future powered by clean, sustainable energy is on the horizon, and hydrogen is poised to be a major player in this intriguing new chapter.
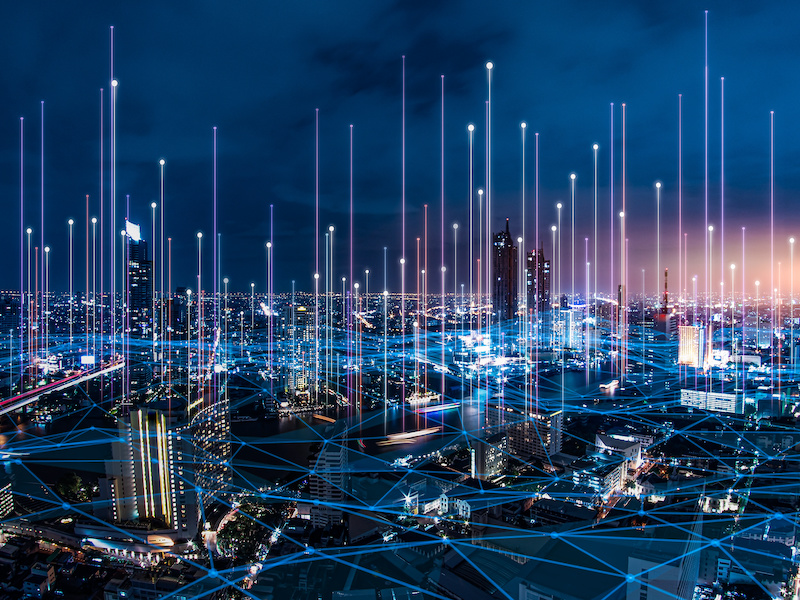
Powering a sustainable tomorrow
We partner with utilities to help them build a more resilient grid and move towards a cleaner, brighter future through
- Smart automation
- Asset image analytics
- DERMS implementation
- Analytics & predictive insights
- Cloud optimization
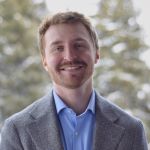